The IceCube Neutrino Observatory
IceCube is an international cosmic neutrino observatory located at the South Pole. The 1 km3 detector is based on a three-dimensional array of 5160 optical sensors (DOMs), which are deployed deep into the polar ice through 86 strings (see figure below). The IceCube experiment is sensitive to high-energy cosmic neutrinos with energies between 100 GeV (100 · 109 eV) and several PeV (1015 eV), although with the denser DeepCore component one can detect neutrinos with energies as low as several GeV. In addition to the InIce detector, located on top of IceCube is the 1 km2 IceTop surface array, which is used to study of cosmic rays.
When a high-energy cosmic neutrino interacts with an ice nucleus within or near IceCube, it will produce energetic charged particles that emit Cherenkov radiation when propagating through the ice. It is this Cherenkov light that is then detected by the DOMs, giving a measure of the energy and the direction of the original neutrino.
As an example, shown in the figure on the right is the track signature of a high-energy muon traversing the detector. Red colors indicate earlier DOM detection times, while green ones indicate later detections; the sizes of the colored spheres are proportional with the amount of photons the corresponding DOM detected. Additionally, the blue line depicts the reconstructed direction of the muon track. This particular event deposited an energy of (2.6 $\pm$ 0.3) PeV in IceCube, and was likely induced by a high-energy cosmic neutrino (more info here).
Cosmic Neutrino Sources
With the IceCube discovery of high-energy cosmic neutrinos in 2013, neutrino astronomy was born. However, to this date, only one source of high-energy neutrinos has been identified. On September 22nd, 2017 a high-energy cosmic neutrino (170922A, see figure below) was observed which led IceCube to issue an alert via the Gamma-ray Coordinate Network (GCN). The IceCube-170922A alert led to a series of follow-up observations in gamma-rays, which identified a strong high-energy photon flux coming from the same direction. In this position on the sky lies the blazar TXS 0506+056, which was flaring during that time (see the section Blazar Flares). As such, the first evidence has been found that the blazar TXS 0506+056 is a source of high-energy cosmic neutrinos. Moreover, this coincident detection of a high-energy cosmic neutrino and gamma-rays marked the entrance of cosmic neutrinos to multimessenger astronomy.
After this coincident detection, the IceCube Collaboration performed an exclusive neutrino analysis on TXS 0506+056. It was found that an excess of high-energy neutrinos was observed from the direction of TXS 0506+056 between September 2014 and March 2015. This provides additional and independent evidence for the blazar TXS 0506+056 being a source of high-energy neutrinos. It should be noted that several members of the IIHE were involved in the above efforts.
However, TXS 0506+056 can not account for the whole diffuse cosmic neutrino flux observed by IceCube. Therefore, many searches are being performed to find the sources of high-energy cosmic neutrinos. There is also a much older mystery, namely which sources accelerate the particles that reach Earth with energies larger than 1018 eV. These ultra-high-energy cosmic rays, which are charged atomic nuclei such as protons $\mathrm{p}$, are more than a million times more energetic than those in the LHC, which will reach 7 TeV (7 $\times$ 1012 eV) in LHC's final stage (corresponding with collisions at a center-of-mass energy of 14 TeV). Their precise origin can not be seen by detectors on Earth since thanks to their electric charge, their path is bent by magnetic fields $\vec{B}$ (see figure below). But in many models, neutrinos $\nu$ (which are electrically neutral) are also produced by these same particles when they interact in the source, together with gamma-rays $\gamma$ (photons). Gamma-rays can however easily be absorbed by matter they encounter on their path. In this case, the only way to identify the source is by means of neutrinos.
At the IIHE, several studies are being performed to identify possible sources of high-energy cosmic neutrinos that could also be responsible for the acceleration of ultra-high-energy cosmic rays. Because of their tremendous energies, these cosmic rays cannot be contained by the magnetic field of the Milky Way, our Galaxy. Their origin thus lies most likely outside of the Milky Way. Therefore, the searches which are described below are all focusing on such extragalactic high-energy cosmic neutrino source candidates. Other searches for neutrinos coming from less distant astrophysical phenomena, such as solar flares and dark matter from the center of the Milky Way and the center of the Earth, are discussed in later sections.
Blazar Flares
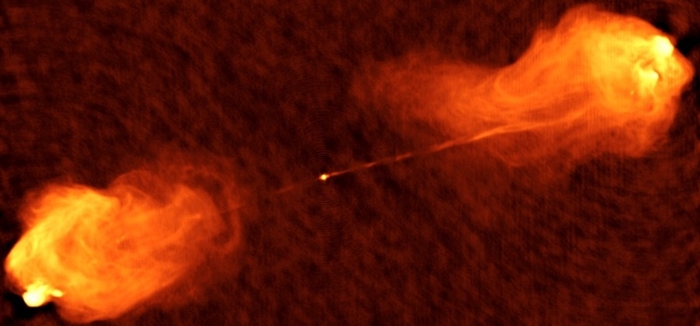
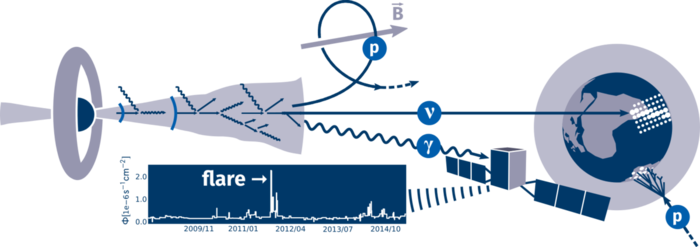
Gamma-Ray Bursts
Gamma-Ray Bursts (GRBs) are one of the few objects which, based on the Hillas argument, are capable of accelerating cosmic rays up to the highest energies ($E=10^{20}$ eV). After being discovered in 1967, multiple satellites (e.g. Fermi and Swift) have been launched to study their properties. These studies have shown that two classes of GRBs exist, those related to the collapse of supermassive stars and those associated with the mergers between two compact objects, at least one of which is a neutron star. This source hypothesis of the latter category was confirmed in August 2017, when LIGO and VIRGO observed gravitational waves coming from a binary neutron star merger.
In addition to photons and gravitational waves, GRBs are also predicted to be sources of neutrinos and cosmic rays. Using data from the IceCube neutrino observatory, searches have been made that looked for correlations between the arrival time and direction of neutrinos and gamma-rays. Observing a neutrino flux coming from GRBs would definitively prove that they are also sources of cosmic rays. The latest prompt neutrino flux constrains by IceCube are shown in the figure on the right (arXiv:1702.06868).
While no statistically significant correlations have so far been found, previous searches have typically been resticted to time windows that are on the order of the duration of observed gamma-ray flashes (1-200 seconds, i.e. the prompt phase of GRBs). Precursor photon flashes up to 3 minutes before the main outburst are however observed in over 10% of all GRBs. As GRB are initially expected to be optically thick, the high particle density at the start of GRBs forms an ideal environment for neutrino production. At the IIHE, we therefore study the possible correlations between the neutrinos observed by IceCube and the precursor phases of GRBs. In addition, the possibility that neutrinos are produced in the aftermath of the prompt phase is also under investigation. With these studies, we aim to discover a neutrino flux coming from GRBs or to constrain the physics models which have been proposed to describe the precursor, prompt and afterglow phases of GRBs.
Previous IIHE Research on Gamma-Ray Bursts
- Lionel Brayeur (PhD, 2015) — Search for High-Energy Neutrinos Associated with Long Gamma Ray Bursts using the IceCube Detector
- Martin Casier (PhD, 2015) — Search for High-Energy Neutrino Production in Short Gamma Ray Bursts with the IceCube Neutrino Observatory
- Dennis Diederix (Master, 2010) — Model Independent Search for Neutrinos from Gamma Ray Bursts with the IceCube Detector
Obscured Sources
In the picture where high-energy cosmic neutrinos are produced by (ultra-)high-energy cosmic rays at their source, one would also expect gamma-rays to originate from the same objects. As such, one could search for neutrinos from bright gamma-ray sources, such as blazars (see the section Blazar Flares). However, although more than 80% of the diffuse gamma-ray flux measured by the Fermi satellite is due to blazars, the contribution of blazars to the diffuse neutrino flux measured with IceCube is strongly constrained. Note that this is not in contradiction with the fact that the first identified source of high-energy cosmic neutrinos is a blazar. The figure on the right shows the Fermi gamma-ray data in red and the IceCube neutrino data in black (see paper). Substracting the blazar contribution from the Fermi gamma-ray flux (full red line) and determining the corresponding neutrino flux (full black line) leads to a deficit w.r.t. the IceCube data.
To resolve this gamma-neutrino tension, at the IIHE the idea was proposed that the cosmic neutrino sources could be opaque to gamma-rays, i.e. that the gamma-rays would be absorbed before escaping their source. Consequently, such an obscured source analysis is being performed on Ultra-Luminous Infrared Galaxies (ULIRGs), of which an example is shown in the image on the right (this example is called the “Toothbrush Galaxy”; note that these are in fact interacting galaxies, which is a typical feature of ULIRGs). These objects are characterised by an infrared luminosity exceeding $L_{\odot}^{12}$ (where $L_{\odot} = 3.83 \times 10^{33}$ erg s-1 is one solar luminosity). This extreme infrared luminosity not only indicates that there is a lot of dust being heated at the source, which could absorb the gamma-rays, but also means that there is a large energy budget available for the acceleration of particles and thus for the production of high-energy neutrinos. Moreover, the interactions of accelerated cosmic rays with the ambient matter could further enhance the neutrino production.
Previous IIHE Research on Obscured Sources
- Giuliano Maggi (PhD, 2017) — Search for High-Energy Neutrinos from Obscured Flat Spectrum Radio AGN using the IceCube Neutrino Observatory
Low-Energy (GeV) Astrophysical Neutrinos
GeV Neutrinos from Solar Flares
Since the end of the eighties and in response to an increase in the total neutrino flux in the Homestake experiment, in apparent coincidence with major solar flares, solar neutrino experiments are trying to identify neutrinos produced during these sudden flashes of energy. To date, no confirmation of an increase in the neutrino rate due to solar flares has been found. Detection of neutrinos from solar flares would open a new window on these phenomena and increase our insight in the underlying physical processes. A new analysis proposed at the IIHE searched for low-energy neutrinos in IceCube in coincidence with observations of gamma-rays detected by Fermi-LAT.
Previous IIHE Research on GeV Neutrinos from Solar Flares
- Gwenhaël de Wasseige (PhD, 2018) — Solar Flare Neutrinos in the Multi-Messenger Era: Flux Calculations and a Search with the IceCube Neutrino Observatory
- Gwenhaël de Wasseige (Master, 2014) — Search for GeV Neutrinos from Solar Flares with the IceCube Neutrino Observatory
GeV Neutrinos from Gamma-Ray Bursts
While TeV neutrinos are predicted as a consequence of the internal shocks in the prompt phase of the GRBs, GeV neutrinos should be produced by neutron and proton collisions following decoupling. A neutrino search in the GeV energy range would therefore be complementary to the existing limits that have been set by neutrino telescopes in the TeV range. Some models also predict the neutrino emission before, or after the prompt phase. In this analysis we searched for an excess of GeV neutrinos in time windows from 3 s to 1000 s around gravitational wave events as possible counterparts of short GRBs.
Previous IIHE Research on GeV Neutrinos from Gamma-Ray Bursts
- Gwenhaël de Wasseige (PhD, 2018) — Solar Flare Neutrinos in the Multi-Messenger Era: Flux Calculations and a Search with the IceCube Neutrino Observatory (Chapter 7)
Dark Matter
Dark matter was postulated as soon as in the 30s by Fritz Zwicky in order to account for the existence of “unseen matter” in the Universe. Even if we have strong observational evidence of dark matter, its nature remains unknown. To date, the most commonly admitted hypothesis is that dark matter is made out of particles, unobserved yet, called Weakly Interactive Massive Particles (WIMPs).
Several approaches are used in order to search for dark matter particles, which can be regrouped in three main categories: direct searches, dark matter production in particle accelerators and indirect searches. Indirect search experiments, such as IceCube, are looking for secondary Standard Model particles produced by the decay or self-annihilation of dark matter. These experiments usually focus on regions where a high density of dark matter is expected, like the Galactic Center. A dense WIMP population is also expected in the center of heavy objects, such as the Sun and the Earth (see figure below).
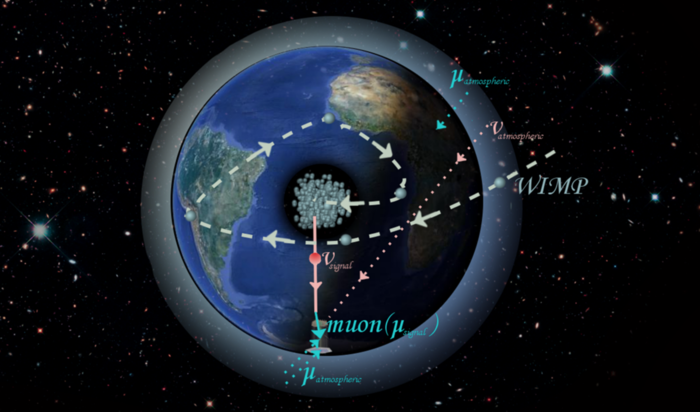
Earth WIMPs
In previous years we performed an analysis on one year of data in search for possible WIMPs annihilating in the center of the Earth (see the WIMPs introduction for some more details). With this, we were able to set upper limits on the WIMP annihilation cross section. But since then, IceCube has spent many years maturing, being tested in a variety of analyses, with many contributions to improve the quality of the data we can use. That's why we are currently working to apply the same method to an extended dataset of multiple years. This should allow us to improve on our previous results.
Previous IIHE Research on Earth WIMPs
- Jan Kunnen (PhD, 2015) — A Search for Dark Matter in the Center of the Earth with the IceCube Neutrino Detector
Galactic Center Combined Search
We are currently performing an analysis to search for dark matter annihilation in the Galactic Center using both the ANTARES and IceCube neutrino telescopes. Limits on the WIMP self-annihilation cross-section in the Galactic Center have already been set by both experiments. By combining their observations, we are expecting to improve these limits for the energy range where the two detectors are comparable, that is to say between 50 GeV and 1 TeV. Another goal of this combined search is to understand the approaches of ANTARES and IceCube for this kind of analysis and develop a unified analysis method.
Previous IIHE Research on Galactic Center Combined Search
- Nadège Iovine (Master, 2017) — Combined Search for Neutrinos from Dark Matter Annihilation in the Galactic Centre using IceCube and ANTARES
Beyond IceCube
SiPMs
Silicon Photomultipliers (SiPMs; see figure on the right) are single-photon-sensitive light detectors. SiPMs are efficient in a cryogenic environment, which makes them a good alternative to photomultipliers (PMTs) in a lot of experiments such as IceCube. They are also robust, relatively cheap, and have a low power consumption.
The IceCube Collaboration is planning to extend the volume of the detector to roughly 10 km$^{3}$ with the IceCube Gen2 project. The idea is also to extend the surface veto, for which SiPMs and scintillator panels are being considered. The goal will be to combine efforts regarding the characterization of SiPMs and measurements of the PhotoDetection Efficiency (PDE).
Radio Extension
At the highest energies, the cosmic neutrino flux drops rapidly and above a few PeV the IceCube neutrino detector runs low in statistics. To probe the cosmic neutrino flux at these energies an even larger volume than the cubic kilometer currently covered by IceCube is needed. Due to its long attenuation length and cost efficient detectors, the radio signal provides the ideal means to probe the cosmic neutrino flux above PeV energies.
At the IIHE, we work on a new, novel, radio detection technique to probe high-energy neutrino induced particle cascades. This is done by actively probing the ionization plasma left behind after the neutrino-induced particle cascade propagates through the ice, through the radar detection method (see figure below). This method, if shown to be efficient, allows us to lower the energy threshold of the currently existing radio detectors searching for high energy cosmic neutrinos which is found around EeV energies.
The efficiency of the radar detection technique is currently under investigation, where several beam test experiments have been performed. Besides the radar detection technique, we investigate a possible cosmic ray background signal given by coherent transition radiation of a high-energy cosmic ray air shower hitting the air-ice surface. This signal, if identified correctly, does not only allow for the on-site calibration of the radio detectors searching for the high-energy cosmic neutrino flux, but would immediately give the on-site feasibility of the detection method.
Theses
Click here to get the list of all IIHE PhD and Master theses on IceCube.
Outreach
At the IIHE, we are closely involved in all outreach activities related to the IceCube experiment. These activities go from the translation of IceCube comics to the making of movies and also to the organisation of events such as the South Pole Experiment Contest. Below a listing is given of all the outreach activities the IceCube group at the IIHE contributes to.
Events
Videos
- IceCube Masterclass 2015 — event video and student testimonials
- Movie on the study of Gamma Ray Bursts
- Q&A on the first cosmic neutrino source — full event and soundbites
- Official YouTube Channel of the IceCube Neutrino Observatory
Other
- IceCube comic — The Adventures of Rosie and Gibbs